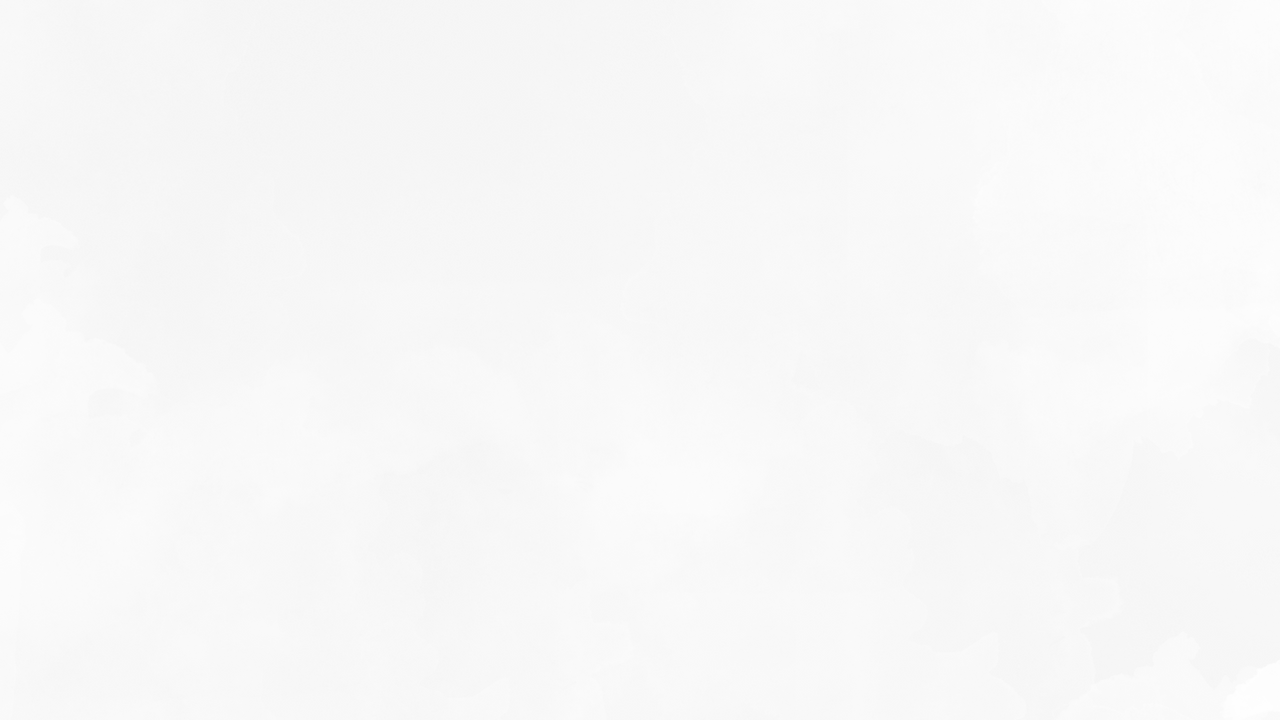
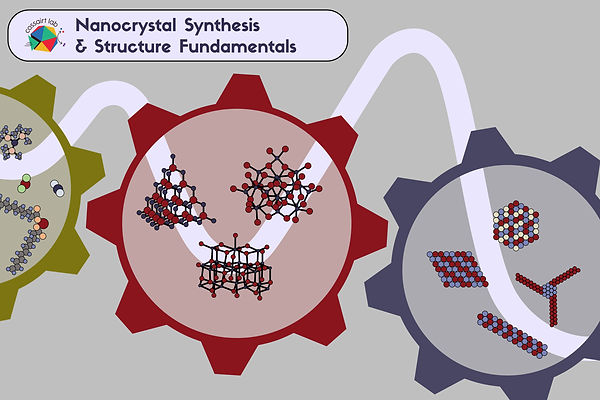
Nanocrystal synthesis and structure fundamentals
Understanding and controlling fundamental nanocrystal synthesis is crucial to efficiently produce high quality nanocrystals including quantum dots and ternary nanomaterials for eventual use in display and lighting, magneto-optical, and charge transport technologies. The Cossairt lab is interested in conversion pathways between atomically precise ‘magic-sized’ nanoclusters, understanding the interplay between nucleation, dissolution, aggregation, and quantized mechanisms of growth. An ongoing theme in the Cossairt Lab is to approach science from multiple perspectives through collaboration, which is realized through our involvement in CSSAS, a center engaged in biomineralization and dynamic hybrid material systems and more. We gain rich insights to study InP QD nucleation and growth with inspiration in and beyond traditional inorganic nucleation theories. Our exploratory research is supported through characterization techniques such as UVvis absorbance, NMR techniques, SC- and P-XRD, and high resolution TEM, allowing unprecedented images of particles as small as 1 nm.
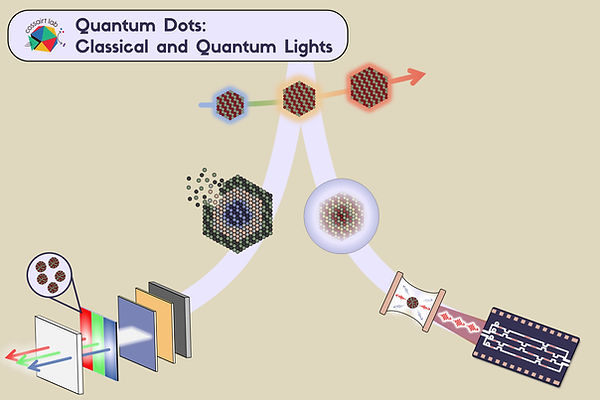
Quantum dot classical and quantum light
Quantum dots are an excellent class of efficient light generators, with important applications ranging from LED lighting to quantum information science. In the Cossairt lab, we collaborate with members of the IMOD center and the MEM-C center to target the generations of both classical and “non-classical” lights from the synthetic perspectives. Through atomically precise surface chemistry, we aim to understand the surface and interfacial structure of InP quantum dots and clusters. Our current efforts include exploring new strategies to build intentional oxide interfaces and rigid ligand networks with designer functionalities to modulate the electronic structure and emissive properties with techniques including X-ray spectroscopy, and LED device fabrication. For effective non-classical or quantum light generation, solid state single-photon sources need to be deterministically positioned and coupled with nano-optical cavities. We aim to leverage advantages of solution processable materials to improve integration of single-photon sources to photonic devices.
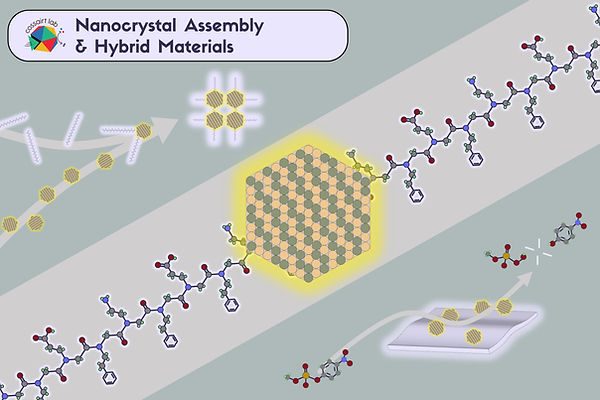
Nanocrystal assembly and hybrid materials
Natural systems have mastered the power of a structure-function relationship, in which organic and inorganic moieties interact to form a hierarchical and discrete arrangement. In the Cossairt lab, we explore hierarchical bioinorganic assembly through inorganic nanoparticles, mainly quantum dots and metal oxides, and sequence-defined poly-N-substituted glycines, known as peptoids. Eventually, we hope to expand this work to create superluminescent materials that have the benefit of solution processability, structural stability, and efficient electronic communication between quantum dots. Additionally, in collaboration with the Pacific Northwest National Laboratory (PNNL), we are investigating the conjugation of metal oxide nanoparticles to sheet-forming peptoids and their catalytic hydrolysis of nerve agent simulants. Our main method of characterization involves transmission electron microscopy (TEM), which allows us to become very intimate with the nanoscale, and visually identify atomic lattices, phase transitions and packing trends, and dispersity.
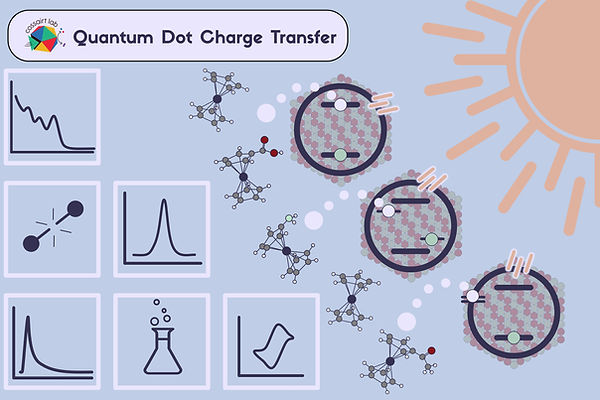
Quantum dot charge transfer
Chemical manufacturing accounts for about 10% of total global energy consumption and 7% of greenhouse emissions. Photocatalysts offer a carbon neutral and mild alternative pathway to perform thermodynamically uphill reactions, by converting solar energy to chemical energy. This conversion of energy often requires extraction of high energy charge carriers. In our lab, we tune charge carrier dynamics through surface chemistry, morphology, heterostructure design, and engineered defects. We measure the effects of our chemical design spectroscopically, photoelectrochemically, and through photocatalytic reactions.

Earth abundant materials for electrocatalysis
Developing earth-abundant alternatives to noble metal catalysts that convert renewables into commodity chemicals and fuels is a critical step towards global sustainability. Transition metal phosphide (TMP) nanocrystals in particular have been shown to catalyze numerous energy conversion and storage reactions, with the hydrogen evolution reaction (HER) being the most well studied. The high activity of TMPs toward HER is attributed to their binary nature, which modulates surface energetics and active site geometry. We hypothesize that the benefits of a binary material can be expanded toward catalyzing complex electrochemical reactions due to the tunable polarity of the surface (i.e., various transition metal and stoichiometric variations of each TMP). This ultimately may assist in heterolytic bond cleavage and adsorption of a variety of intermediates required in complex electrochemical reactions. Ongoing research efforts in our group aim to synthesize various types of transition metal-based nanocrystals (TMPs and beyond) and study their surfaces for applications in hydrogen-relevant electrocatalysis beyond HER (e.g., nitrate reduction, alcohol oxidation, etc). We are building a rigorous understanding of the surface chemistry of these nanocrystals via electrochemical studies in non-aqueous and aqueous conditions to identify design principles that underlie catalyst activity. We are also using X-ray spectroscopy to probe the corrosion stability of nanocrystals in order to understand and prevent degradation detrimental to electrocatalysis.